The role of insulation systems in limiting heat transfer
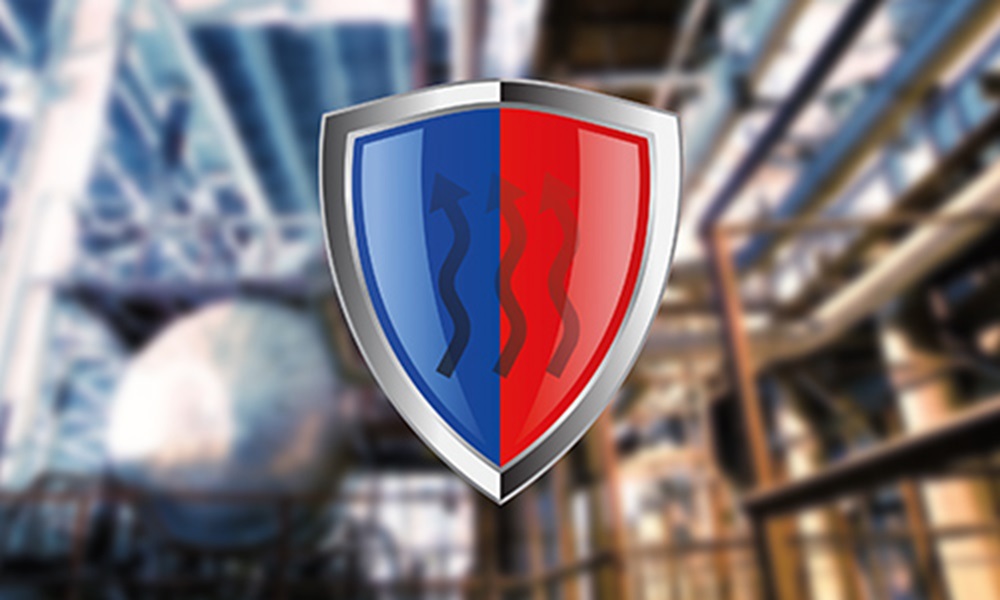
The role of an insulation system is to provide thermal resistance under sometimes harsh environmental and service conditions. Ironically, these are the very same conditions that can degrade an insulation system to the point where problems with process control, personnel protection, equipment damage and increased operating costs can occur. The key to having systems continue to operate in a safe and efficient manner is to choose an insulation material that can deliver unchanged thermal performance under demanding environmental and service conditions.
Heat transfer 101
Heat can be transferred in three different ways - conduction, convection and radiation. Conduction is the transfer of energy (heat) within a material or between two bodies in physical contact. Convection is the transfer of heat by movement of parts of a liquid or gas within the material because of differences in temperature, density. etc. within the material. Radiation is the transfer of energy (heat) from a higher-temperature body, through space, to another lower-temperature body without warming the space between the two bodies.
These terms are used when talking about heat transfer in real world applications and are defined in accordance with ASTM C168 and ISO 7345. This discussion will primarily focus on heat transfer by conduction.
All materials have a property called thermal conductivity also known in certain industries as “k” value or “λ” value. Thermal conductivity is defined as the rate at which heat passes through a specified material. It is expressed as the amount of heat that flows per unit time through a unit area with a temperature gradient of one degree per unit distance. Standard units of measurement and reporting are in terms of W/m•K or Btu-in./hr-ft²-°F. For uniform materials, thermal conductivity is not dependent on the area, thickness or shape of the material; however, the total quantity of heat transmitted is dependent upon these factors.
In addition, thermal conductivity is dependent upon temperature and is often reported along with mean temperature of the material over a range of possible application temperatures. It is important to understand how thermal conductivity of material may change with temperature, as this will have significant design considerations for insulation systems. Standard test methods for determining conductivity are specified in ASTM C177, ASTM C518 and EN ISO 13787.
In addition to thermal conductivity or “k” value, a few other terms are frequently used when discussing heat transfer of materials:
Thermal resistance (R) is a heat property of a body or assembly measured by the ratio of the difference between the average temperatures of two surfaces to the steady state heat flux in common through them (the rate of heat flow per unit area of one surface which must be identified). The “R” value for a material can be found by dividing the thickness of a material by its “k” or thermal conductivity value.
“R” value is mostly commonly seen in consumer building materials and construction.
Thermal conductance (C) is the property of a body or assembly measured by the ratio of steady state heat flux in common between two definite surfaces (time rate of heat flow per unit area of one surface which must be identified) to the difference between the average temperatures of the two surfaces. The inverse of the “R” value (or 1/R) is equal to the “C” value.
Thermal transmittance (U) is the overall coefficient of heat transfer—the ratio of the steady state heat flux from the surroundings on one side of a body, through the body, to the surroundings on its opposite side (time rate of heat flow per unit area of a surface which must be identified) to the temperature difference between the two surroundings.
WHAT CAN AFFECT THE THERMAL CONDUCTIVITY OF AN INSULATION MATERIAL?
Moisture infiltration
Moisture infiltration is the prime threat to thermal efficiency. Moisture absorption can increase heat flow and subsequently operating costs. It can also be detrimental to product output quality and quantity.
Examples include excessive boil-off, production interruption due to viscosity changes, destruction of equipment and possibly plant shutdown.
Other issues that can manifest are problems related to corrosion and personnel protection issues because of increased surface temperatures on hot systems.
Insulations containing liquid water exhibit thermal conductivity values up to 3 times greater than when dry.1
Under freezing conditions, conductivity may increase even more as the thermal conductivity of ice is 4-6 times higher than the thermal conductivity of water.
Recent studies on open cell insulation materials have shown that a 1% increase in moisture content can result in a 23% increase in thermal conductivity.2
Depending on the porosity of an insulation, heat flow can increase up to 300 percent with a presence of just 20 percent (vol.) moisture.3
1 Cremaschi et al., 2012a; Wilkes et al., 2002
2 A M Gusyachkin et al., 2019
3 Weiwei Zhu et al., 2014
Moisture can enter an insulation directly as absorbed water through gaps in joints and sealants, openings in jacketing, or from the inside out via pipe or vessel leakage.
Also, it is important to note that moisture can enter an insulation before, during or after installation.
An even more significant source of moisture penetration and soaked insulation is diffusion from water vapor, which subsequently condenses as a liquid or ice in below ambient or cold processes.
This phenomenon is particularly common in many open celled or otherwise permeable insulation which rely on jacketing to prevent or slow moisture intrusion.
Some closed-cell materials, such as commonly specified cellular plastics will allow slow moisture vapor diffusion when a vapor pressure differential exists between one side of the product and the other. Moisture laden air then accumulates in cells from which the blowing agent has diffused. This can lead to wet and underperforming insulation systems which is why these materials are often protected by vapor barriers or retarders.
These materials can be susceptible to structural distortion, imperfect seals or damage caused by mechanical abuse. On cryogenic systems, for example, even a small pinhole breach can result in ice formation in a matter of days.
Consequently, in many applications, it is more critical that an insulation has a low vapor transmission rate than a low “out of the box” thermal conductivity.
Material aging
Insulation degradation due to aging or thermal drift, results from “outgassing,” or gas diffusion through cell walls of plastic foam insulation materials. Gas transmission takes place due to the difference in gas concentrations between the inside and the outside of the cells and temperature-induced internal and external pressure differences. Temperature extremes, exposure to chemicals and radiation can exacerbate ageing over time. The result can be that the actual thermal conductivity of a material in application is significantly higher than published values resulting in a dramatic difference in actual thermal efficiency v. design efficiency. Cellular plastic foam insulations like polyisocyanurate, polyurethane and phenolic foam are particularly prone to these influences and tests have shown that after two years, polyisocyanurate samples averaged 22 percent above their labeled k factor.4
Other studies have shown that aging and loss of thermal efficiency can continue for as long as 20 years after the insulation is installed.5
4 Kenneth E. Wilkes et al., 2001
5 Kim Jin-Hee et al., 2020
Liquid Chemical Absorption
The thermal conductivity of spilled, leaked or even atmospheric chemicals may add to the increased thermal conductivity of already wet insulation. Additionally, chemical attack may physically destroy the insulation or at least degrade thermal efficiency and mechanical strength. Foamed plastics and open cell insulation materials are susceptible to a loss of thermal performance due to chemical absorption. This is particularly true when the insulation has previously been weakened by moisture intrusion.
Certain systems rely on waterproofing agents to protect the insulation on high-temperature systems. Even if effective waterproofing is in place, it will not retard absorption of simple hydrocarbons. In fact, these waterproofing agents can be destroyed by such liquids enabling the insulation material to absorb liquid chemicals which can lead to the decrease of thermal performance and increased fire risks.
THE FOAMGLAS® CELLULAR GLASS INSULATION SOLUTION
It is clear that liquid absorption and retention are among of the most destructive elements for an insulation system. Therefore, it is highly beneficial to specify an impermeable insulation material that does not have to rely on an external vapor barrier to keep the moisture from infiltrating the insulation.
FOAMGLAS® cellular glass insulation is an all-glass, 100% closed-cell material that eliminates the risk of moisture (vapor) intrusion into the insulation material.
Even after total immersion in water, the only measurable moisture found on FOAMGLAS® insulation is that which adheres to its surface cells.
Moisture penetration and material aging can cause a chronic loss of thermal efficiency in other open celled or otherwise permeable insulation materials. FOAMGLAS® insulation is not susceptible to gas diffusion and does not age over time, it provides constant thermal efficiency throughout the life of the system. This minimizes the need for insulation replacement and helps support designs aimed for lower long-term, life-cycle costs.
The all-glass composition, and the fact that there are no binders or fillers makes FOAMGLAS® insulation one of the most chemically durable insulation materials available. This helps to ensure that the mechanical and thermal performance of a FOAMGLAS® insulation system will not be affected by possible external or internal chemical attack while mitigating fire and structural corrosion risk to pipelines and equipment.