The importance of dimensional stability and its influence on the performance of an insulation material
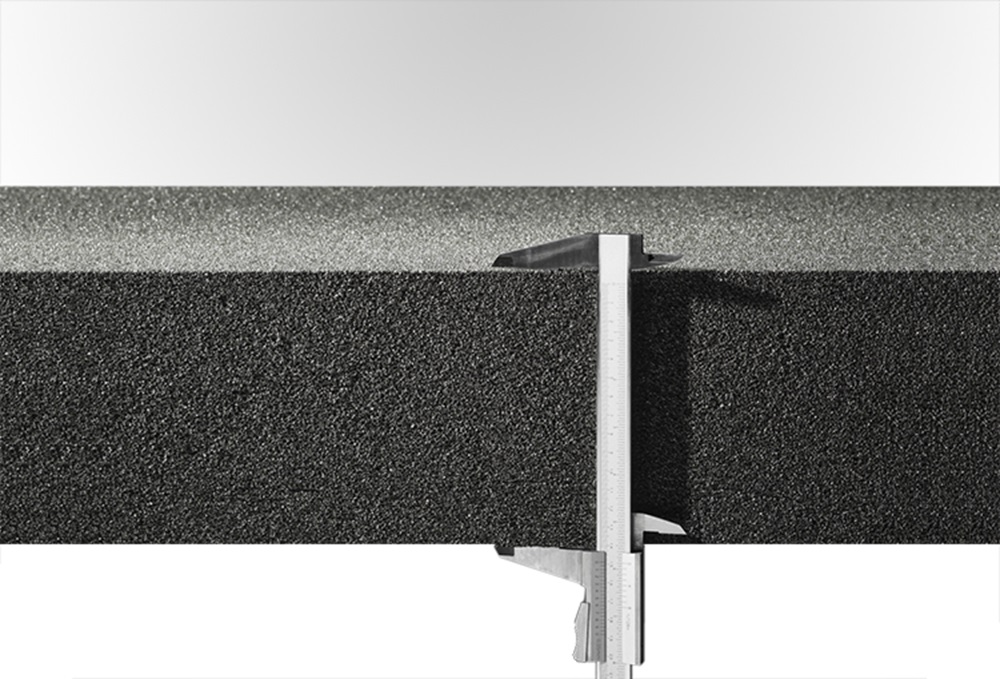
Insulation performance and system integrity is directly related to the dimensional stability of the insulating material. Poor dimensional stability can cause swelling, expansion, shrinkage and buckling of the insulation. This behavior can eventually lead to thermal bridges between insulation and equipment, coating/waterproofing breaches, and, most critically, unpredictable insulation performance.
FACTORS THAT AFFECT DIMENSIONAL STABILITY
Reversible Changes at Low Temperatures
The rate of reversible, dimensional change—the coefficient of thermal expansion/contraction—exhibited when the temperature of an insulation material changes is most often related to its chemical composition. In general, organic materials have a greater coefficient of thermal expansion than inorganic materials.
Organics, such as plastic foams, display coefficients five to ten times greater than those of metal substrates. This can result in shrinkage resulting in open joints that create a thermal short circuit pathway permitting convection and allow for substantial heat gain which reduces the overall thermal efficiency. Joints that have been sealed against water intrusion also can become compromised allowing moisture to penetrate the system
To counter these thermal movements that take place with organic foams, expansion and contraction joints must be foreseen in order to mediate the movements of the insulation parts following the thermal contraction and relaxation to prevent the creation of thermal gaps.
Cellular glass insulation exhibits a predictable, minimal, reversible coefficient of thermal contraction close of that of carbon or stainless steel and concrete. Therefore, little relative movement occurs at the insulation joints during system temperature cycling.
Expansion / contraction joints are costly in terms of materials, labor, and time. Low differential movement between the piping and the insulation material can result in systems designed and installed with fewer expansion / contraction joints.
Reversible Changes at Moderate Temperatures
At elevated temperatures, the problem is reversed; the high thermal expansion coefficient of organic foams can lead to warping and buckling, putting severe stress on weather barriers and vapor retarders. This could put the integrity of the insulation system at risk and negatively influence the thermal performance over time.
Phenolic foam and other plastic foams can showcase dimensional changes at elevated temperatures when heated up to 130 °C (266 °F) or higher.
In contrast, cellular glass insulation remains stable since it is well matched in expansion coefficient to typical steel piping and equipment.
Irreversible Changes at High Temperatures
At high temperatures, inorganic insulation must be used. However, as temperatures rise and metal pipes and vessels expand, some insulation materials actually shrink. Insulation materials such as perlite and calcium Silicate can exhibit shrinking of up to 2% when exposed to their upper use temperature range.1
The combination of shrinking insulation and expanding piping and equipment can lead to open joints and cracks which can cause thermal short circuits and serious damage to weather barriers.
Cellular glass insulation has a reversible coefficient of expansion similar to metals. Therefore, reducing the potential for issues caused by differential shrinkage or expansion.
Other Irreversible Changes
Aging
Permanent dimensional changes can have many causes, including aging of the insulation material. The term ‘aging’ refers to the decay of the thermal insulating properties and dimensional stability of rigid closed-cell foams due to the outward diffusion of the low conductivity blowing agent and the inward diffusion of higher conductivity air constituents
This leads to post-production shrinkage of plastic foams (particularly polyurethane), possible blistering with foamed-in-place PUR, and outgassing of foaming agents that eventually can result in shrinkage of up to 2%.
On cold systems, low density polyurethane’s in-cell gases can condense, break down cell walls and lead to insulation collapse.
Temperature and Humidity
Moisture can penetrate some insulation materials during its storage, transportation or installation and it can become trapped by vapor barriers or vapor stopping. In addition, malfunctioning vapor barriers can admit moisture before startup or during operation.
Combined with temperature changes, a significant alteration of insulation dimensions can occur. A similar problem can occur when plastic foams, which have a relatively high permeability, are subject to the ingress of water vapor due to a faulty or inadequate vapor barrier being installed or otherwise being subjected to on-site damage.
In all these cases, the insulation material is not only in contact with high humidity but also subject to changes in the temperature of the insulated system and the outside ambient temperature.
Also, solar radiation can increase the temperature by more than 75 °C (135 °F), dependent on the climate and geographic situation.
Under the combined influence of temperature and humidity, plastic foams can suffer a remarkable change of dimensions. Shrinkage, warping or bowing of the insulation are the result.
Polyurethane at 70 °C (158 °F) and 85% relative humidity shows an irreversible expansion of 3% (one manufacturer states that even greater changes are possible).
On the other hand, under similar conditions phenolic foams can shrink up to 2%. Polyisocyanurate is affected by humid aging. Its rapid, permanent warping puts severe stress on external vapor and weather barriers.
With cellular glass insulation, service and testing have again proven that it remains dimensionally stable under extreme humidity.
Temperature and Loading
Load on an insulation at elevated temperatures is a potential source of dimensional change with possibly severe consequences.
Therefore, foamed plastics manufacturers publish temperature-related load recommendations.
The combination of high temperature resistance, rigidity and the high compressive strength of cellular glass insulation however, creates excellent dimensional stability under a wide range of temperatures.
The FOAMGLAS® Insulation Solution
Compared with other insulation materials, especially plastic foams with a 5 to 10 times higher coefficient of contraction, it is understandable why FOAMGLAS® insulation provides the solution for insulation systems with variable temperature conditions.
Its coefficient of thermal contraction that is close of that of steel and concrete helps to ensure that virtually no relative movement occurs at the insulation joints during system temperature cycling.
Several tests, plus practical experience over many years, shows that no irreversible dimensional changes with FOAMGLAS® insulation have been observed. Even for temperatures of about 400 °C (752 °F) FOAMGLAS® insulation does not suffer from shrinkage compared to other inorganic insulation materials.
High humidity has no effect as testing and practical experience has shown that FOAMGLAS® insulation remains dimensionally stable when it is in contact with humidity or water.
During tests at 20 °C (68 °F) and 95% relative humidity, no changes could be observed which is why FOAMGLAS® insulation is also often a preferred insulation material when the ambient conditions involve high humidity.
The influence of extreme temperatures in combination with heavy loads does not affect FOAMGLAS® insulation’s performance helping to ensure excellent dimensional stability under load at a wide range of temperatures.
This is also one of the reasons why it is the preferred tank base insulation material by tank builders all around the world to support their cryogenic, cold and high temperature tanks.